Investigating Mitochondria Transfer for Glioblastoma
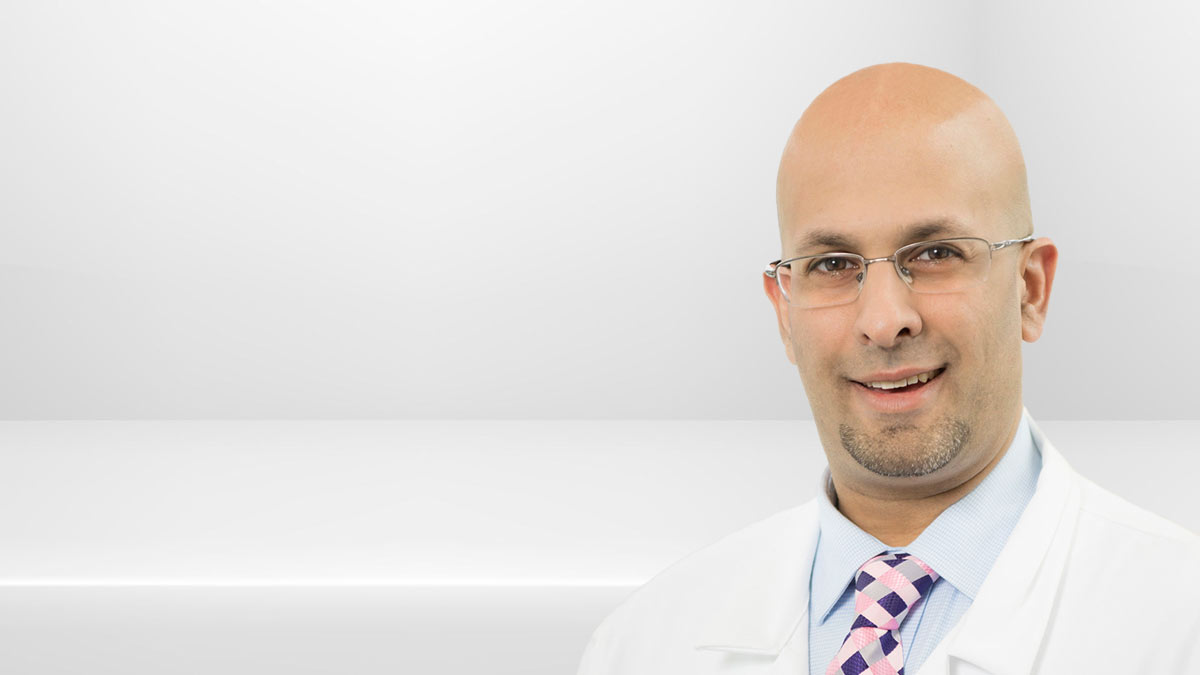
The Cancer Advances podcast is joined by Justin Lathia, PhD, Melvin H. Burkhardt Endowed Chair for Neuro-Oncology Research, Scientific Director of the Rose Ella Burkhardt Brain Tumor and Neuro-Oncology Center, and the Vice Chair of the Department of Cardiovascular and Metabolic Sciences at Cleveland Clinic's Lerner Research Institute to talk about his study on mitochondria transfer for glioblastoma. Listen as Dr. Lathia shares the complexity of glioblastomas, the challenges in treating it, and the potential of mitochondria transfer as a communication process.
Subscribe: Apple Podcasts | Podcast Addict | Buzzsprout | Spotify
Investigating Mitochondria Transfer for Glioblastoma
Podcast Transcript
Dale Shepard, MD, PhD: Cancer Advances, a Cleveland Clinic podcast for medical professionals, exploring the latest innovative research and clinical advances in the field of oncology.
Thank you for joining us for another episode of Cancer Advances. I'm your host, Dr. Dale Shepherd, a medical oncologist here at Cleveland Clinic, directing the Taussig early cancer therapeutics program and co-directing the Cleveland Clinic sarcoma program. Today I'm very happy to be joined by Dr. Justin Lathia, the Melvin H. Burkhart endowed chair for neuro-oncology clinical research and vice chair of the Department of Cardiovascular and Metabolic Sciences in Cleveland Clinic's Lerner Research Institute. He's here today to talk to us about investigating mitochondria transfer for glioblastoma. So welcome, Justin.
Justin Lathia, PhD: Thanks, Dale. It's great to be here.
Dale Shepard, MD, PhD: So, give us a little bit of an idea, what do you do here at Cleveland Clinic?
Justin Lathia, PhD: That's a good question. It's a little bit more than drinking coffee and telling jokes. I run a laboratory-based program where we're very interested in understanding the complexity of glioblastoma, which is the most common primary malignant brain tumor. And we do this in a variety of ways. We do this in very close collaboration with our colleagues at the Brain Tumor Center. So these are neurosurgeons, neuro-oncologists, neuropathologists, and other caregivers where we work to get human tissue from patients that are treated here, we bring it into the laboratory, try to understand what's going on, use them to build models, and we ask basic science questions.
And then what we try to do at the end of these exercises is trying to understand, can we take what we learned and bring it into an early phase clinical trial? So, we've done this a couple of times, where we've taken laboratory laboratory-based discoveries, which were partly utilizing human samples here, and then spun them back out into early phase clinical trials. And I think it's really important, because these tumors have a very poor prognosis. You can count on one hand the number of FDA approved drugs in the last 50 years. The standard of care was set in 2005. And if you think about the advances in biology and biomedical science, we're really lagging behind. So, we're trying to close that gap.
Dale Shepard, MD, PhD: So, it's fantastic you have the bedside to bench to bedside kind of approach here.
Justin Lathia, PhD: Exactly.
Dale Shepard, MD, PhD: Glioblastomas, just give us a brief overview. You mentioned the severity of it. What are they, and maybe a little insight, why are they so hard to treat?
Justin Lathia, PhD: Yeah, so what are they? Embarrassingly enough, I can't give you a very precise answer. I'll do my best. So, these are cells that form a tumor in the brain, so it's a primary brain tumor. What's still up for debate is what cell in the brain was the initial cell or what we call the cell of origin. So that's still up for debate, but once these tumors present, like ovarian cancer, actually, they present at a really late stage. So, it's not like you can screen it genetically. You can't screen blood biomarkers. These are tumors that present late. And then once they present, obviously they're growing in a very intricate organ, so the surgeons will take out as much of it as they can. And it's followed by radiation and chemotherapy.
So why are these tumors so hard to treat? The cells themselves are very plastic, so they're very adaptable. So, they can resist therapy. They're almost all shapeshifters. They can change into different cell states. I mean, crazy stuff like they can use different fuels. So, there's this idea in cancer about targeting metabolism. Well, there's a problem here, because these cells are metabolically plastic. They can use things like glucose, they can switch to fatty acids. They're very plastic in that way. They also have an elevation in developmental programs. So developmental programs are absolutely essential to build organs, but these programs are hijacked by these cells. There's also challenges with the cells invading the entire brain. So, if you have a bunch of cells going everywhere and your MRI is only telling you where the focal region is, I think that remains a challenge. So, it's likely a combination of plasticity, as well as the infiltrative nature that makes these tumors so difficult to treat.
Dale Shepard, MD, PhD: And when we talk about cancers, most other cancers, they spread to other parts of the body, whereas glioblastomas tend to stay in the brain.
Justin Lathia, PhD: That's correct.
Dale Shepard, MD, PhD: And a lot of the treatments we use, we have a hard time getting into the brain.
Justin Lathia, PhD: Yeah, thanks for bringing that up, Dale. I should have mentioned that one of the other major challenges is this anatomical structure that many people may have learned about maybe in medical school or graduate school called the blood brain barrier. This really is allegedly, not allegedly, this is set up to protect the brain from a variety of systemic insults and toxins, but it also makes it more difficult to get very, very potent agents that work systemically into the brain.
Dale Shepard, MD, PhD: There's been no shortage of ways to try to disrupt blood brain barrier and things like that, a little promising, but then ultimately don't work so well. So, the things you're talking about, the more plasticity and things are probably a lot more important.
Justin Lathia, PhD: I do want to highlight though, some work going on here by some colleagues in neurosurgery and neuro-oncology where they're starting to use ultrasound to temporarily disrupt the blood brain barrier. And I think my optimism is set in that, that may be a modality that could be leveraged, because it's super safe, from what we can tell, and it's a temporary way for us to get more chemo, more targeted agents into the brain. So, I would say stay tuned for that.
Dale Shepard, MD, PhD: All right. We are going to talk about mitochondria transfer.
Justin Lathia, PhD: Yeah.
Dale Shepard, MD, PhD: Tell me, what exactly is that all about?
Justin Lathia, PhD: Okay, so we should start from the origins. One of the tenets of our laboratory is we're very interested in what we call the languages and dialects of cell communication. So, I would say my background is more in developmental neurobiology and biomedical engineering. And when you put those two things together, we tend to view cancer a little bit differently. It's more than just a series of mutations. It's more than just a ball of cells growing in the brain. What it is, it's almost like an integrated circuit. And what we have been focusing on is trying to understand how these circuits function, because if we understand that, I think it allows us to build better therapies. Now, how did we get on mitochondria? So, there are examples where mitochondria, which are very important for cell growth and homeostasis, can actually transfer from one cell to another.
Now if you look in the brain at catastrophic situations like a stroke, it actually has been demonstrated that astrocytes, one of the major support cells in the brain, can almost try to resuscitate neurons by donating whole mitochondria. So, this is theoretically possible, but no one's really looked in glioblastoma. And again, being interested in the languages and dialects of communication, this could be a potential way for cells to communicate. And that's really the basis of that project. I mean, I'm happy to take you through the high-level details.
Dale Shepard, MD, PhD: Yeah, that'd be great.
Justin Lathia, PhD: Okay. So unfortunately, it's tough to do this for human patients. So, we ended up using a lot of mouse models. And we played a lot of tricks where we would use mice where every mitochondrion in that mouse was labeled, let's just say red. We would put green tumor cells in and then we would take the green tumor cells out later and see if they had red mitochondria. And that showed us that communication was possible. We also were able to separate these tumor cells into tumor cells that had mitochondria versus didn't have mitochondria from the donors. And then we did a lot of cells biology-based experiments to show that when a glioblastoma cell gets mitochondria from its environment, it is actually more prone to grow. It is more prone to utilize developmental programs. It has a reorganization in part in its metabolism, which allows it to really continue to grow without exhaustion. So that's quite interesting. And in terms of the cell types, we screened a bunch of cell types in the brain, and it seemed like the astrocytes were the biggest donor. So that's sort of the background on that.
Dale Shepard, MD, PhD: So, when we think about astrocytes, and you mentioned strokes, and clearly there may be some advantage to try to regenerate neurons, is that a process that is kind of an ongoing maintenance of neuron health process as well? So, I guess thinking downstream, as a medical oncologist, I'm always what I can target kind of guy. If you were to target that transfer process and try to stop that, would you have negative effects on normal functions in the brain, or too early to know?
Justin Lathia, PhD: Short answer is it's too early to know but let me tell you what we're thinking. So, we're very aware of the need to understand what we like to think about as tumor specific. Again, for someone who is trained in developmental neurobiology, I'm keenly aware of the importance of maintaining neural integrity. So, the thing that we're doing a lot of comparative analysis, so what are transfer mechanisms that astrocytes or frankly any other neural cell uses when it's talking to a tumor versus when it's talking to someone else? And I think once we get our grasp on that, we can do what we normally do, where we do a lot of drug screening.
And our initial strategy is usually using FDA approved drugs. If we can use FDA an approved drugs and say, "Hey, just so you know, this antifungal that was developed for this actually stops this process," it allows us to quickly translate this into an early phase clinical trial. I'm not implying we're there yet. I mean we still have to understand the tumor specificity. We've got to do the drug screens properly, we've got to work up the molecules, we've got to ensure they get into the brain, but the general strategy would be exactly that. Is there a specific dialect that the tumor cells are using that normal cells aren't?
Dale Shepard, MD, PhD: And at this point your research, you've shown there's a particular growth associated protein that we think is an important factor. Is that right?
Justin Lathia, PhD: That's right. So, we were trying to understand, you can show the communication occurs, you can show the consequence of the communication, but scientifically, we really wanted to know what we call the molecular mechanism. How is this happening? And we actually found that. So, the protein's called GAP 43, so growth associated protein 43. It's actually very important early on in development of the brain for neurons, actually. And we found that actually GAP 43 was really the bridging protein between the astrocyte and the tumor cell that facilitated the mitochondria transfer. And when we altered the expression of GAP 43, we actually slowed down the transfer. So that gave us our first hint as to whether it is likely that GAP 43 is partially responsible for this process.
Dale Shepard, MD, PhD: So, GAP 43 is on the astrocyte. Is there a similar process that we can identify on the glioblastoma cells themselves?
Justin Lathia, PhD: Yeah, so actually GAP 43 is expressed by both cells.
Dale Shepard, MD, PhD: Okay.
Justin Lathia, PhD: So that's actually important to know. So now moving forward, when you think about therapy, we're trying to understand the GAP 43 expression in other neural cell types. And there are some really nice tricks that we can leverage that we could potentially target only the glioblastoma cells and alter GAP 43 only in them. But again, it's early days there and it's a lot of action on the whiteboard right now in the lab as to how to think about this.
Dale Shepard, MD, PhD: It's an important step.
Justin Lathia, PhD: Absolutely.
Dale Shepard, MD, PhD: Do we think that this process, the mitochondrial transfer, plays into maintaining glioblastoma and promoting growth or initiation of glioblastomas, or what do we know about that process?
Justin Lathia, PhD: Yeah, our studies really highlight growth and progression. In terms of initiation, this is something I think that's been challenging the field. We don't have the best models to do this. We're diving into this right now and trying to use newer models, but it's very difficult to tell what the initiator is when the preclinical models you use are you take a bunch of cells that you grew in a dish, and you put them into the brain of a mouse. You have been the initiator. So, I think understanding those early stages is going to be very important.
Dale Shepard, MD, PhD: And without knowing, as you mentioned before, how do you track that to know, I guess? What other interesting factors have you seen in terms of metabolic factors or anything else right now that looks to be controlling glioblastoma growth?
Justin Lathia, PhD: I think the thing that's been staring us in the face for years is the metabolic plasticity. So, I think trying to get a better handle on that's going to be important. Glioblastoma in particular, I think there's some reports out there about, for example, the ketogenic diet. I'm sure that on this podcast or on this program, people have at least talked about it. I mean, as a medical oncologist, I'm sure when you see patients, they may ask you, "Doc, can I change my diet in some way?"
Dale Shepard, MD, PhD: All the time?
Justin Lathia, PhD: It's an easy to modify thing. So, I think there is an opportunity to alter metabolism. And the other thing to think about and again, a lot of work's come out of the Cleveland Clinic on cardiovascular disease. Well, what about the microbiome? What about the bugs in my gut? How can they be altered in some way? And these are things we're thinking about. But again, it's a lot of whiteboard action in the lab right now. But if you said, "Hey, Justin, 10 years from now, what do you think you'll be working on?" I think these will definitely be key components of what we're working on.
Dale Shepard, MD, PhD: When we think about other solid tumors, there's a lot of thought that's gone into treating not just the tumor itself, but the tumor microenvironment, things like that. Cell signaling seems like an important part of that. Are there other factors that come into play with glioblastomas as well?
Justin Lathia, PhD: Yeah. I mean, I think this initial observation of mitochondria transfer, I'd be remiss if I didn't also mention that right around the time we published this paper, we had colleagues at the University of Utah. So, the group was run by Mina Roe Johnson, and we collaborated with Mina's group. Same thing happens in breast cancer, but in breast cancer, it's the infiltrating macrophages that do this. So, I think there's a lot going on. And viewing the tumor as more than just a mutated ball of cells is going to be very important. And I think if you even look at pancreatic cancer, that's a great example of where this idea of the tumor microenvironment, the cancer associated fibroblast, is a key driver in how these tumors are growing. They're becoming aggressive and they're resisting therapy.
Dale Shepard, MD, PhD: You mentioned a couple of times a lot of whiteboard thoughts about how this works. I guess the way of the world in research is you have a great idea, it's a great idea, unless you have the support to turn that great idea into research. Tell a little bit as this is released, it is going to be right around the time for VeloSano. Tell us a little bit about how fundraising efforts, how VeloSano has been able to support some of your work.
Justin Lathia, PhD: Sure. Yeah, so you really have highlighted the paradigm for biomedical research, where someone like me spends a lot of time writing grants, trying to get these ideas funded. And really the ideas have to be very robust and very well vetted to be competitive at the government level. So, the National Institutes of Health, and for us mainly the National Cancer Institute, is a huge funder of biomedical research, but the funding rate's low. It's, I don't know, could be as low as single digits some years. So how do you get over that hump? And when you have a crazy idea, when you have an idea that is really high risk, a lot of the larger funding bodies are risk averse. And that's where VeloSano changes the game for us. It puts us in a position to say, "I have this crazy idea. I'm going to do a focused set of experiments."
We call these go, no-go. If they don't work, we've learned something, but if they do work, oh my God, this could change the way we think about biology and medicine. And I think having VeloSano has been a catalyst. The one thing I like to say is since the inception of VeloSano, our lab's been lucky enough to get some of these crazy ideas funded. I feel like the majority of the work in the lab right now has VeloSano's fingerprints on it. VeloSano, for example, has helped us spin out an early phase clinical trial because we were interested in how tumor cells communicate with immune cells, and we found a series of therapies, one of which we were able to take into the clinic and treat patients here at the Cleveland Clinic. And again, a lot of these ideas would not make it past sort of the initial stages if it wasn't for philanthropy. So, I think that's why pilot funding, seed funding, funding of high-risk ideas, which generally won't happen at the upper levels, is really important for us to change the way science is done and medicine is practiced.
Dale Shepard, MD, PhD: And for a disease like glioblastoma where really the standard therapy was in 2005 is really, really important. And it's going to take those crazy ideas, as you put it, to make a difference.
Justin Lathia, PhD: Yeah. And I would be as bold as to say that if you look at any advance in science or medicine in the last 20 years, those initial stages, people will sort of the initial reaction is, "This is crazy. It won't work." Someone like me who spends a lot of time in the lab, we have all these textbooks sitting on our walls, sitting on our bookshelves, and our job is to basically try to fill in the gaps or demonstrate that something in that textbook isn't actually quite accurate. It's a huge challenge.
Dale Shepard, MD, PhD: Well, you're doing really important research on a really serious disease, and I appreciate you joining us today and giving us your insight.
Justin Lathia, PhD: It's a real pleasure, Dale. Thanks again.
Dale Shepard, MD, PhD: To make a direct online referral to our Taussig Cancer Institute, complete our online cancer patient referral form by visiting clevelandclinic.org/cancerpatientreferrals. You'll receive confirmation once the appointment is scheduled.
This concludes this episode of Cancer Advances. You'll find additional podcast episodes on our website, clevelandclinic.org/canceradvancespodcast. Subscribe to the podcast on iTunes, Google Play, Spotify, SoundCloud, or wherever you listen to podcasts. And don't forget, you can access real-time updates from Cleveland Clinic's Cancer Center experts on our Consult QD website at consultqd.clevelandclinic.org/cancer. Thank you for listening. Please join us again soon.
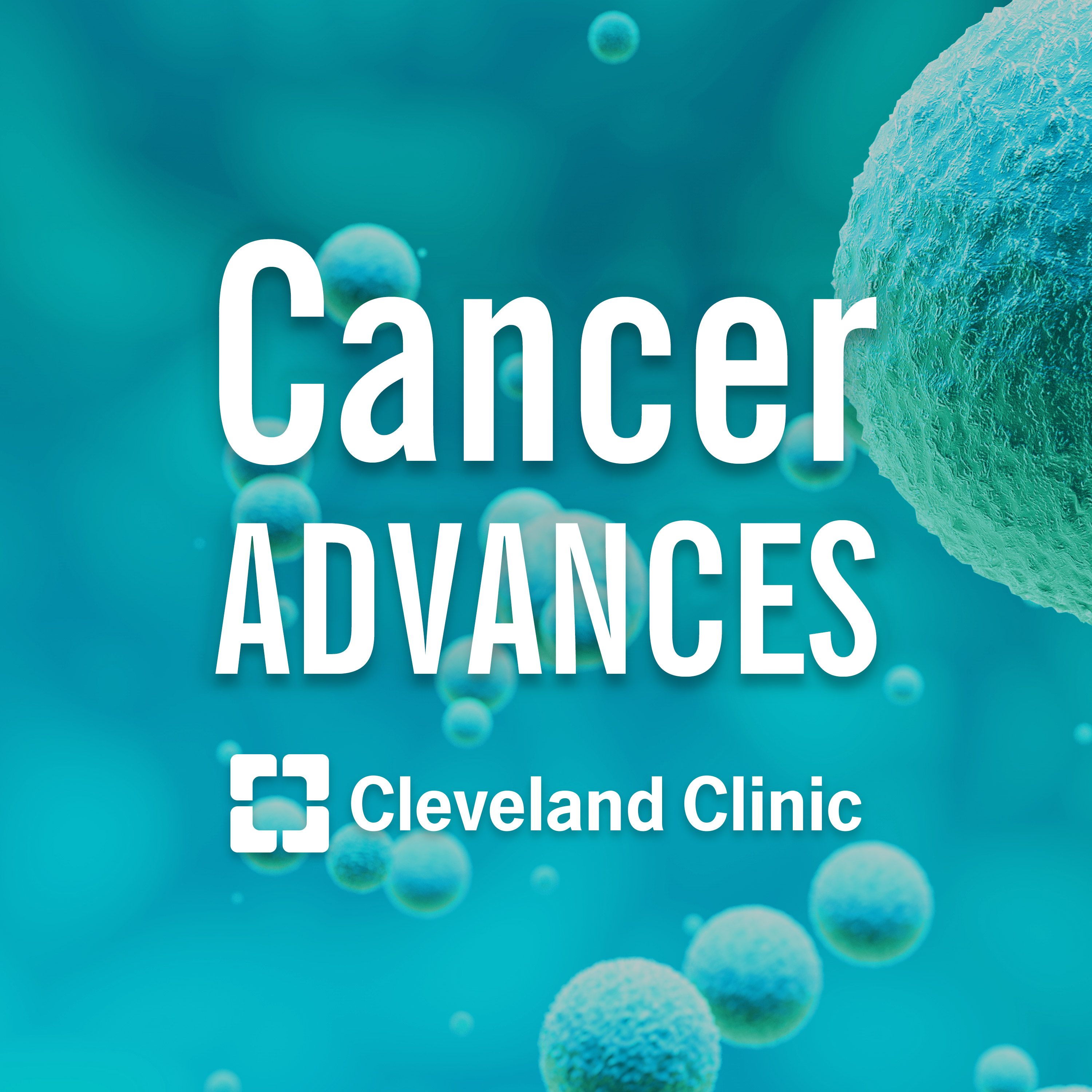